In the past ten years I have worked with four international collaborations and several universities and physics laboratories in two different research fields in physics: gravitational waves and particle physics. I like to think of myself as a lucky physicist. Indeed, during my physics education I managed to see the observation of two milestones in modern physics: gravitational waves and the Higgs boson. These observations have reshaped our understanding of gravity and of the origin of mass in the Universe. Theorists and experimentalists who significantly contributed to these discoveries were awarded the Nobel Prize in Physics in 2013 and in 2017, respectively.
In this page you can find a short description of my research activity in the past years:
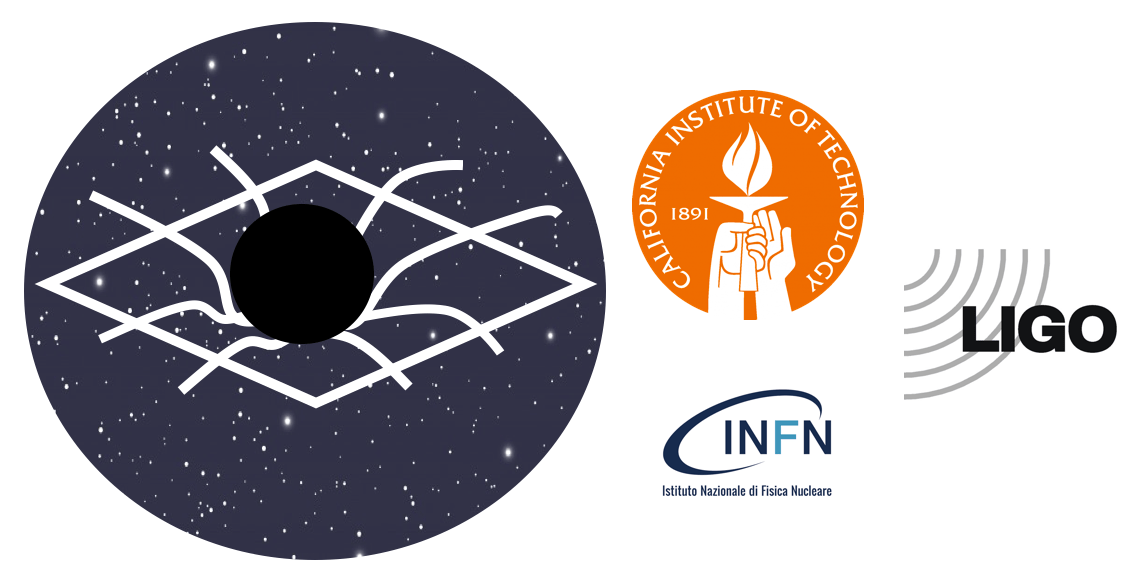
GRAVITATIONAL WAVES
On the 14th of September 2015 the Laser Interferometer Gravitational-wave Observatory (LIGO) detector observed a waveform compatible with the predictions of general relativity for the emission of gravitational waves for the merger of a pair of black holes and the subsequent formation of a heavier black hole. I worked several months in the LIGO laboratories of the CALifornia Institute of TECHnology (Caltech) to better understand some dissipation processes occurring in the isolation system of the LIGO detector. Do you want to know more on my research? Have a look at this LIGO public document.
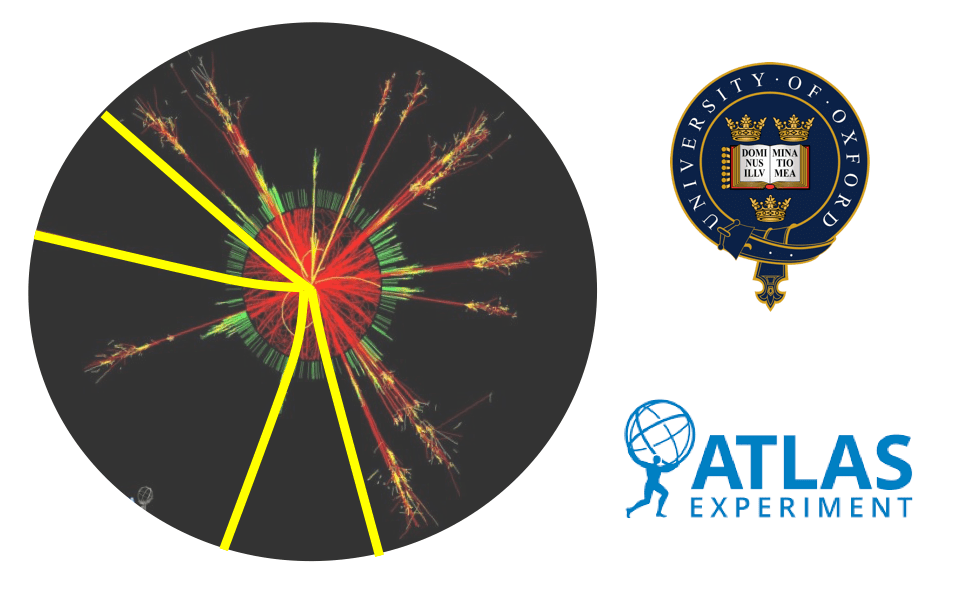
THE HIGGS BOSON
After almost fifty years of predictions and theoretical speculations, in 2012 the ATLAS and CMS experiments of the Large Hadron Collider (LHC) particle accelerator of CERN observed a new particle, the Higgs boson. This particle played a key-role in the evolution of the universe right after the Big Bang giving mass to all the fundamental constituents of matter. The Higgs boson is an unstable particle, it decays (i.e. disintegrates) into other particles. My DPhil research with the university of Oxford was one of two best measurements worldwide of the Higgs boson total width, a property related to the Higgs boson disintegration rate. Do you want to know more on my research? Have a look at the ATLAS paper reporting on this interesting physics result.
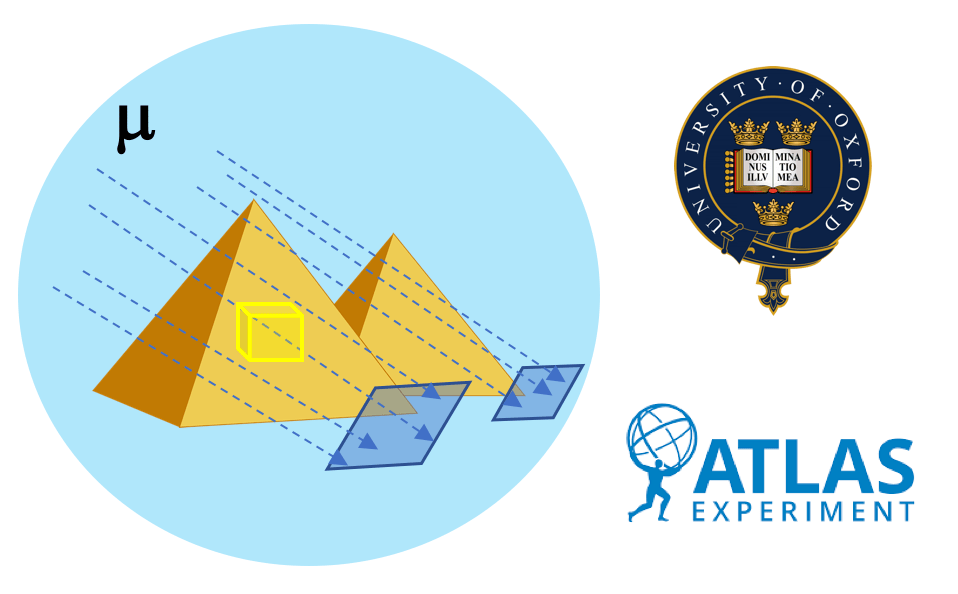
MUON PHYSICS
Muons (μ) are elementary particles similar to the well-known electrons, but 200 times heavier. As a consequence of this heaviness they can penetrate matter far more deeply than electrons, even through kilometres of rock. This can be exploited for muon radiography or muography based on cosmic muons. In 2017 a muography of Khufu’s Great Pyramid at Giza revealed a big chamber, without any excavation. At the LHC, muons are of key importance to study some of the most interesting topics, as the Higgs boson. My DPhil research with the university of Oxford also included some studies and results in muon physics, which allowed ATLAS to measure the Higgs boson mass at the level of 1.9 permille in 2018. Do you want to know more on my research? Have a look at the ATLAS publication reporting on my muon studies and at the ATLAS paper on the Higgs boson mass.
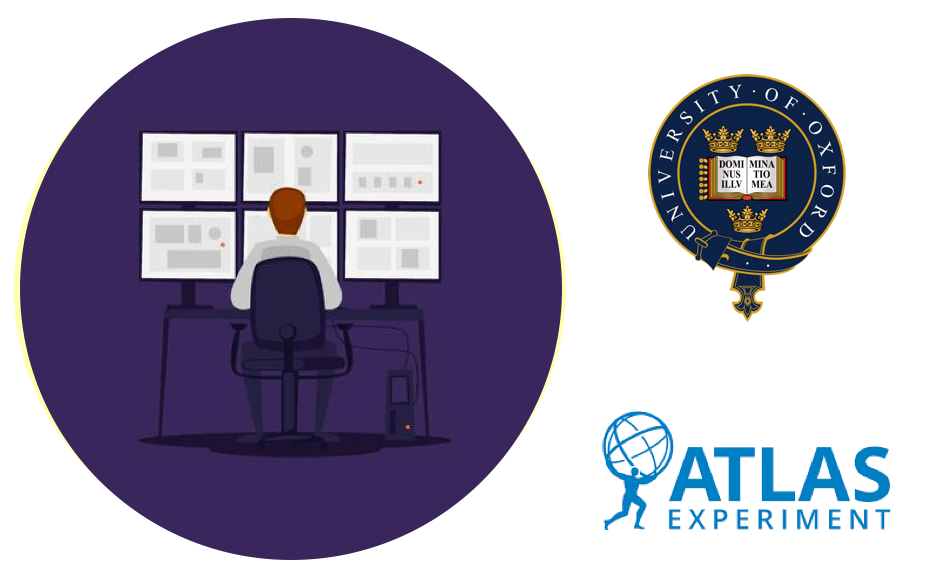
DETECTOR MONITORING
The ATLAS experiment is the largest detector ever constructed by mankind. It’s a huge cylinder, 46m long and 25m in diameter. It’s placed in a cavern 100 meters underground. It is about half the volume of the Notre Dame Cathedral in Paris and it weighs 7,000 tonnes. The detector is instrumented around the collision point, where proton collisions take place with protons traveling at speeds up to 99% the speed of light (300,000 km/s). These collisions reproduce what physicists believe happened a few fractions of second after the Big Bang. ATLAS is like a baby, when you are taking data you need to check the experiment 24 hours a day / 7 days per week. In the ATLAS Control Room there are always at least 6 people monitoring data-taking operations and a Shift Leader, who coordinates and supervises all the shifters’ activities. I was lucky and honoured to serve as the last Shift Leader of the ATLAS Run 2 physics program on the 2nd of December 2018. We celebrated the moment with a cake and some sparkling wine.
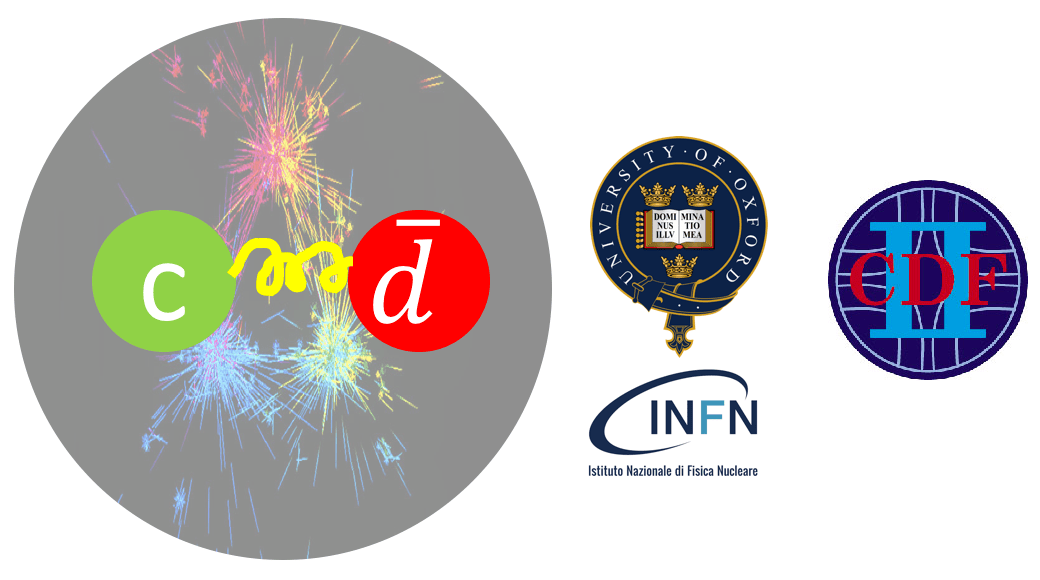
CHARM PHYSICS AND THE STRONG INTERACTION
All the matter in the Universe is composed by two types of fundamental particles, leptons and quarks. A proton is composed of 2 up quarks and 1 down quark (uud). Similarly, a D+ particle is composed solely of two quarks, a charm quark and an antidown quark, as shown in the icon of this section. These quarks are held together via the strong interaction, which acts within the nucleus. The study of the D+ production, especially at low energy, can help us understand how a charm quark can associate with an antidown quark via the strong interaction to make up a composite particle. As a member of the CDF collaboration, I measured the production of the D+ particle at low energy at the Tevatron machine of Fermilab in the USA. Do you want to know more on my research? Have a look at the CDF paper reporting on this interesting physics result.
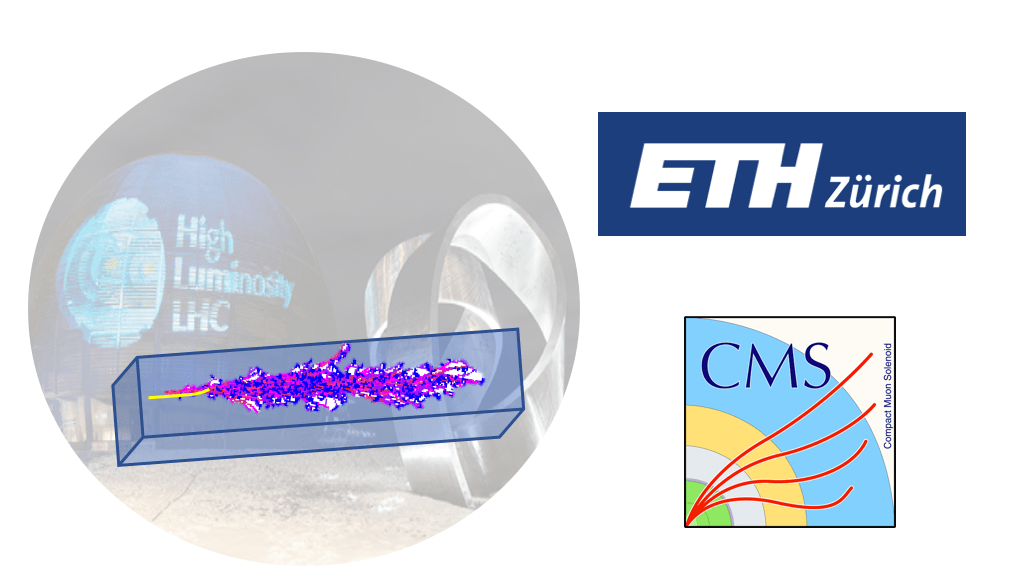
ELECTROMAGNETIC CALORIMETER
In 2024 the LHC machine and its detectors will be extensively upgraded. In the subsequent high luminosity LHC (HL-LHC) era the machine will allow the ATLAS and CMS experiments to collect 30 times the data we collected so far. Significant detector upgrades will be needed to cope with the higher level of radiation. CMS is one of the two major LHC experiments. If ATLAS is about half the volume of the Notre Dame Cathedral in Paris, CMS weighs 14,000 tonnes, more than the Eiffel Tower. Among the various CMS sub-detectors, a special place is reserved to the Electromagnetic calorimeter, the detector able to reconstruct and “see” electrons and photons, where photons are the quantum of the electromagnetic field … what you name light. I currently work with the CMS collaboration and ETH Zürich on the electromagnetic calorimeter. I have a leading role in the current detector operations, software maintenance and detector upgrade for the future HL-LHC machine.
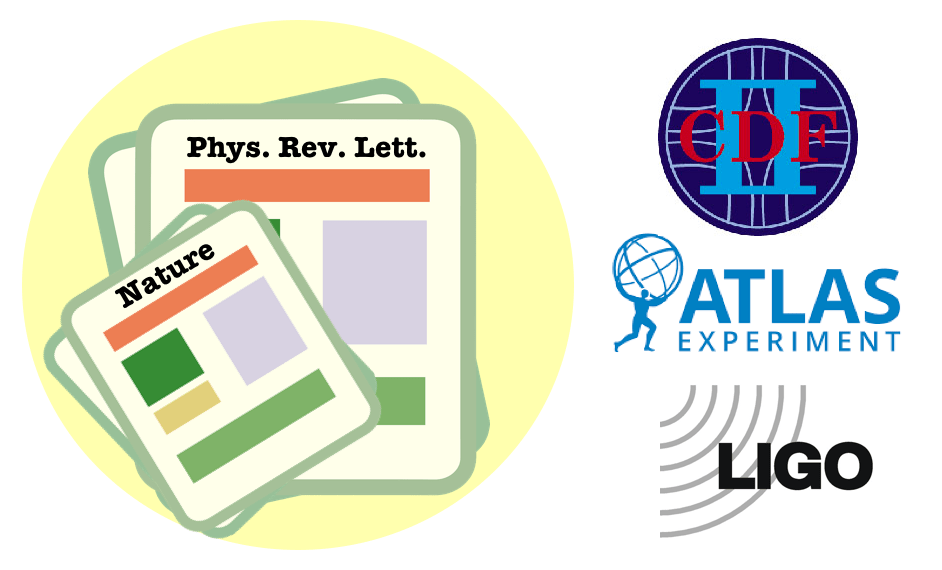
PUBLICATIONS
As a member of the CDF Collaboration’s author list I am co-author of more than 50 papers. As a member of the ATLAS Collaboration’s author list I am co-author of more than 300 papers. The list includes 7 conference publications. The complete list is available here.
Below is a selection of the most important results I have worked on:
- “Constraints on off-shell Higgs boson production and the Higgs boson total width in ZZ → 4l and ZZ → 2l2v final states with the ATLAS detector”, Phys. Lett. B786 (2018) 223-244;
- “Measurement of the D+-Meson Production Cross Section at Low Transverse Momentum in proton-antiproton Collisions at √s = 1.96 TeV”, Phys. Rev. D95 (2017) no. 9, 092006;
- “Validation of the muon momentum corrections for the ATLAS simulation using the Y→µµ channel based on 36.5 fb-1 collision data collected in 2015 and 2016″, ATL-PHYS-PUB-2019-018;
- “Search for heavy ZZ resonances in the l+l–l+l– and l+l–vv final states using proton proton collisions at √s = 13 TeV with the ATLAS detector”, Eur. Phys. J. C78 (2018) no. 4, 293;
- “Search for an invisibly decaying Higgs boson or dark matter candidates produced in association with a Z boson in pp collisions at √s = 13 TeV with the ATLAS detector”, Phys. Lett. B776 (2018) 318-337.
- “Comparative study of material to be used in a geometric anti – spring (GAS) filter for gravitational waves detectors”, LIGO Public doc P1000071-v1.
Click the cover page to access the full text.